Superconductivity and bonded Electrons
When metals are made very, very cold, electrons will bind magnetically to each other. When bonded, they stop being magnetic and expel all external magnetic fields. The simplest bonded electrons are "Cooper Pairs".Normally electrons are magnetic and are called spin 1/2 particles with two states, up and down since they normally go up or down in a magnetic field (commonly called a fermion). When paired up, electrons no longer react to magnets and behave as a spin 0 particle (commonly called a boson).
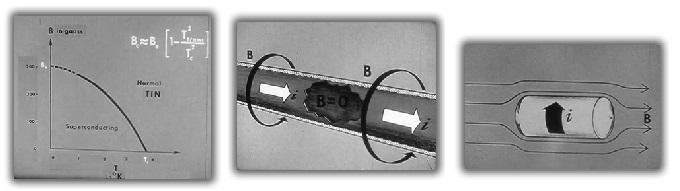
- The temperature that a substance becomes supercontuctive, depends on any external magnetic field that it is within. For tin, with no magnetic field, it becomes supercontuctive at about 3.8°K, in a 300 gauss field, tin will never become a superconducter.
- The cold causes heavier protons to seize up and to stop virtually all motion and the bound electrons to be stuck in place. All extra electrons that are normally conductive, end up on the surface of the material. Electrons flow only on the surface of the material (about 200 Angstoms deep) and corresponding magnetic fields are established.
- A tin tube, supercooled in an external magnetic field, will establish a current flow (electrons) around the the tube. The current flow will establish counter-acting magnetic field (not shown here), that forces the external magnetic field out of the tin tube.
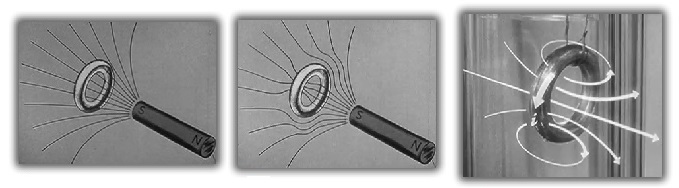
- A normal ring of tin, when exposed to a magnetic field, allows magnetic flux to flow in and through the tin ring.
- As the ring is super-cooled, the magnetic flux lines are forced out of the tin itself. No current is induced at this stage, but the flux lines inside the ring are "stuck" with no way to escape as long as the tin ring is super-cooled.
- As the magnet is removed, the magnetic flux remains trapped within the tin ring. The trapped magnetic flux induces a current flow around the ring. The current flow causes the tin ring to act as a magnet. The magnetic property of the ring has been observed to persist for months at a time forcing the conclusion that there is no resistance to the current flow.
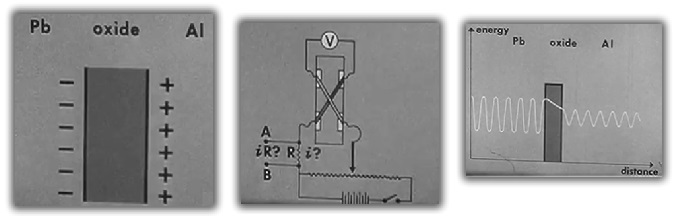
- Lead is used to examine the properties of electrons "bound" to each other. Aluminum is left to oxidize and a lead strip is laid overtop to form a capacitor like structure with oxide between two conductors.
- A circuit is build to allow variable voltage on the two sides of the oxide barrier. Normally a current could not flow through the oxide, but if the oxide is thin enough, electrons are able to "tunnel" across.
- Waves represent the tunnelling process, where the wavelength (width) represents the electron energy and the amplitude (height) represents the number of electrons.
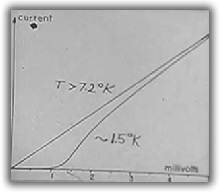
Above 7.2°K, electrons first stablize between the two types of conductors then flow across the barrier with a current directly proportionally to the voltage difference across the oxide.
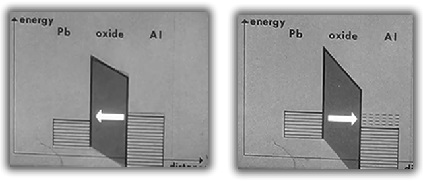
Below the lead superconducting transition temperature of 7.2°K, an energy gap exists. At low voltages, no electrons can flow due to the attractive bond between all the electrons. As the voltage difference is increased, the electron-electron bonds break and the electrons begin to flow across the barrier.
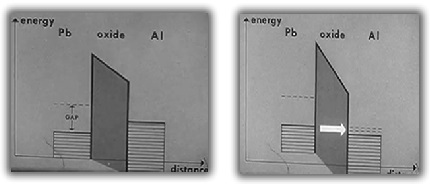